July 2025
This month’s issue of ASHRAE Journal includes topics such as how ASHRAE Standard 62.2 addresses mitigating the risk of long-range transmission of infectious aerosols, the energy savings and health benefits of wet-bulb economizers, and whether assessing risk for flammable refrigerants in air conditioning systems hits the right note.
Features:
How Wet-Bulb Economizers Can Save Energy, Support Health Through Humidification
Chemistry, Regulatory, & Performance Aspects of Antimicrobials for HVAC&R Components
Technology Award: Using Heat Pumps and Solar Energy to Optimize a Multipurpose Residential and Office Building
Technology Award: Energy Conservation and Optimization of Hang Seng Bank Headquarters
Technology Award: Former Power Plant Transforms into STEM Learning Facility
Standing Columns:
Guest Column: Compliance with CAN/ULC-S1001 Standard
Infectious Aerosol Control Column: Infectious Aerosol Control in Standard 62.2
Refrigeration Applications Column: Risk
HVAC Fundamentals Column: Beyond DOAS: Smarter Solutions for Greater Value
ACCESS ARTICLES THROUGH TECHNOLOGY PORTAL
ASHRAE Journal' Podcast
Tune into conversations between leading ASHRAE experts as they discuss what HVAC engineers need to know to design better systems and grow the HVAC&R industry. Listen
Members-Only Free Access to Archived ASHRAE Journal Articles
ASHRAE Technology Portal provides access to ASHRAE Journal. ASHRAE Journal articles provide peer-reviewed "need to know" information and thoughtful insight into the wide range of topics that influence building and system design, installation and operation. The complete archive of ASHRAE Journal issues starting from January 1997 is available. ASHRAE members can download ASHRAE Journal articles in PDF format for free.
Members-Only Free Access to Archived ASHRAE Journal Articles
Non-members can purchase features from the ASHRAE Bookstore. Or, Join ASHRAE!
Digital Journal
The digital Journal is identical to the printed version.
The fully searchable document contains an interactive table of contents with ads linked to advertisers’ Web sites. The digital Journal is available to all ASHRAE members.
View the digital Journal.
AHR Mexico
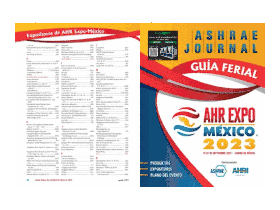
AHR Expo®-México 2023, que se celebrará del 19 al 21 de septiembre en Ciudad de México, México, es un lugar clave para que los fabricantes muestren sus productos y tecnologías más recientes. La guía de la feria de este año incluye la lista de expositores, el plano de la planta e información sobre productos que seguramente será una herramienta a utilizar durante todo el año.
View the digital ShowGuide.
ASHRAE Journal''s Show Daily
Stay up-to-date with ASHRAE Journal's coverage of the 2025 AHR Expo, including the latest information on the 2025 Innovation Awards. Read more
Digital Journal ASHRAE Journal's Official Product and Show Guide for the 2025 AHR Expo
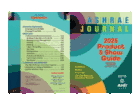
View the digital Show Guide
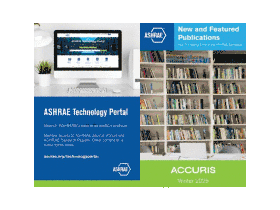
2025 Winter Mini Publications Catalog
View the catalog
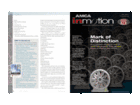
AMCA inmotion
In the 2024 edition of AMCA International’s award-winning magazine: a look inside AMCA’s Certified Ratings Program; maintaining optimal data-center environments with dampers and louvers; combatting heat stress with large-diameter ceiling fans; direct-drive and gear-driven motors; burdens posed by the soon-to-be-finalized regulation for fans and blowers.
View AMCA inmotion
Email Newsletters
HVACR Industry newsletter
The HVAC&R Industry is available to anyone interested in staying up-to-date with the latest news in the HVAC&R industry. In The HVAC&R Industry you'll get:
Current news in the HVAC&R industry
New developments, meeting schedules and other ASHRAE information
A lead story that investigates breaking industry news and a feature story that takes an in-depth look at an industry topic in every issue
Quick links to ASHRAE's online technical information, member-oriented content and other site features:
SUBSCRIBE CURRENT ISSUE ADVERTISE
ASHRAE Journal Newsletter, eSociety, and Insights
ASHRAE Journal Newsletter |
ASHRAE Insights |
ASHRAE Insights Archives |
ASHRAE Journal Newsletter connects today’s technology trends with relevant peer-reviewed content. It is emailed to ASHRAE members on the second and fourth Tuesday.
|
ASHRAE Insights publishes the latest news on research, standards, publications, and more. Insights is emailed to all members on the third Wednesday of each month.
|
ASHRAE Insights Archives provides access to earlier issues with articles of interest to members, including Chapter News, Obituaries, Membership Advancement and other news. Digital archived issues are available back to 2008. Click the Archives tab from the main screen of the current issue.
|
Read archived ASHRAE Journal Newsletters, eSociety, and Insights (requires member login to access)
|
Departments
Access to This Month's ASHRAE Journal Articles
View This Month's Featured Article Excerpts
Indexes to Past Years of ASHRAE Journals
View Indexes
Services
Submit Editorial
ASHRAE Journal welcomes editorials submitted by HVAC&R Professionals. Please see the links below for more information.
Instructions to Authors
Submitting News & Product Information
Submit a Letter to the Editor
View ASHRAE Journal's Submission Guidelines
Subscribe / Advertise
ASHRAE Journal Subscriptions
Purchase enterprise access for your company or library
Advertising